Titled “A piezoelectric, strain-controlled antiferromagnetic memory insensitive to magnetic fields”, a research by the team of Prof. Liu Zhiqi from the School of Materials Science and Engineering was published online in Nature Nanotechnology on January 7, 2019. It adopts piezoelectric strain controlled by electric fields to achieve non-volatile regulation of the spin axis and resistance of antiferromagnet (AFM) MnPt, producing an AFM memory that is fully operational in strong magnetic fields up to 60 T and features low energy consumption.
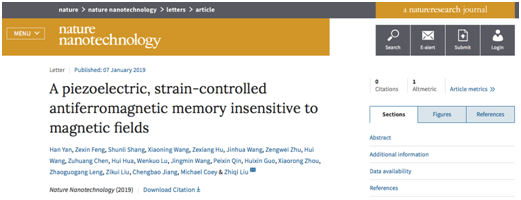
Recent years have witnessed the rapid development of AFM spintronics as a cutting-edge research topic in the field of magnetic materials. Compared with the ferromagnetic materials now used in hard disks, AFM materials have two advantages in terms of information storage: being stable against external magnetic fields (or, to be more specific, against the data loss caused by demangnetization) and increasing the speed of writing data more than a thousand-fold.
The key of AFM spintronics is to control the spin states of AFM materials effectively. Regarding this issue, early studies use the spin–orbit torque produced by electric current to achieve the rotation of the spin axis. However, in strong magnetic fields, the strong Lorentz force will deflect the current and greatly influence the effect of writing and erasing data, which means that the advantage of AFM materials to be magnetic field-resistant can hardly be brought into play.
In a previous research published in Nature Electronics, the effective control of the spin structure of an antiferromagnet has been achieved by the team of Prof. Liu Zhiqi using piezoelectric strain produced by electrical fields. Based on this research, they grow antiferromagnet MnPt films on ferroelectric PMN-PT substrates, and the piezoelectric strain on PMN-PT can effectively change the direction of the AFM spin axis in MnPt (Fig. 1). The resistance of MnPt changes due to the anisotropic magnetoresistance effect in antiferromagnets, leading to different states that can be used to store the binary codes of “0” and “1” (Fig. 2).
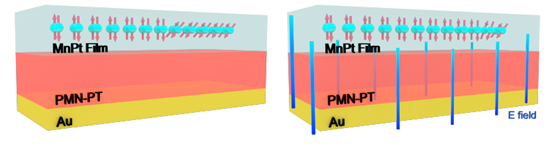
Fig.1 Schematics of the AFM spin axis distribution in MnPt films under the control of electric fields
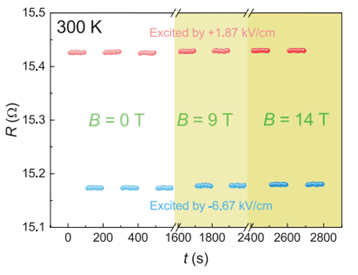
Fig. 2 The high- and low-resistance states written into the AFM memory based on the MnPt/PMN-PT heterostructure
Data writing and erasing by the piezoelectric strain do not involve currents. Even in strong magnetic fields (9 or 14 T), data writing and storage are not influenced at all (Fig. 2). In other words, hard disks made from this kind of device can operate normally in strong magnetic fields.
Furthermore, the team of Prof. Liu Zhiqi collaborated with the team of Prof. Zhu Zengwei from Wuhan National High Magnetic Field Center of Huazhong University of Science and Technology to test the response of AFM MnPt in a 60 T (1.2 million times the strength of the Earth’s magnetic field) magnetic field. According to the results of their experiments, the resistance of MnPt barely varies (~0.1%) because of its strong AFM coupling (Fig. 3) under this condition, which means that this memory will not suffer from demangnetization even in ultrastrong magnetic fields and proves the extraordinary advantage of AFM materials.
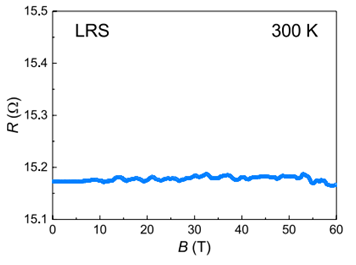
Fig. 3 The resistance variation of the MnPt-based AFM memory under a pulsed magnetic field up to 60 T
Based on the control of the AFM spin axis in MnPt films by piezoelectric strain, the team has also produced an AFM tunnel junction, whose tunnelling anisotropic magnetoresistance reaches ~11.2% at room temperature (Fig. 4). Since previous AFM tunnel junctions can hardly work at room temperature, this is undoubtedly a step forward and has significance for the development of high-density memory applications.
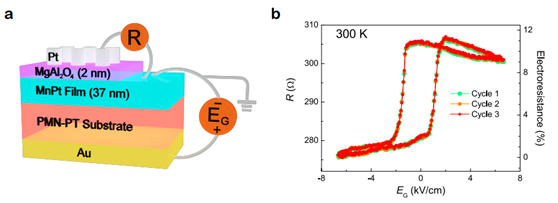
Fig. 4 Piezoelectric strain-controlled room-temperature AFM tunnel junction
According to reviewers, a key point of this research is that the resistance of the AFM material is very stable in ultrastrong pulsed magnetic fields.
The research received great support from Wuhan National High Magnetic Field Center of Huazhong University of Science and Technology, whose experiment facility of strong pulsed magnetic field is the only one in Asia that can provide magnetic fields up to 90T. It was also supported by the National Natural Science Foundation of China (NSFC) on the Science Foundation Ireland–NSFC Partnership Programme, the Excellent Young Scientists Fund of NSFC and Beihang University under the Ten-Thousand Talents Program for top young talents.
Yan Han and Feng Zexin, both graduate students of the School of Materials Science and Engineering, are co-first authors of the research. Guidance was provided by Prof. Michael Coey, a world-renowned expert in magnetics and part-time professor of Beihang University, and Prof. Jiang Chengbao of the School of Materials Science and Engineering. The theoretical calculations were performed by Prof. Liu Zikui and Dr. Shang Shunli of the Pennsylvania State University.
The research article:
https://doi.org/10.1038/s41565-018-0339-0
Reported and reviewed by Xiao Jie
Edited by Jia Aiping
Translated by Li Mingzhu