On May 15, the team led by Professor Cheng Qunfeng and Academician Jiang Lei published their collaborative work titled “Ultrastrong Graphene Films via Long-Chain π-Bridging” in Matter, a journal from Cell Press and a sister to Cell. Prof. Cheng serves as the corresponding author and Wan Sijie, a PhD student enrolled in 2014 is the first author of the research paper. Beihang is the only corresponding institution.
It is of great research importance to convert abundant low-price natural graphite into high-performance graphene films at room temperature. Currently, it has been demonstrated that low-temperature oxidization is an effective strategy to exfoliate graphite into high-quality graphene oxide (GO) nanosheets. In addition, hydroiodic acid (HI) reduction at room temperature is a widely used method to efficiently reduce GO into reduced GO (rGO). The method of chemical vapor deposition (CVD) is proved to be feasible for fabricating graphene films on a large scale. However, it still remains a technical challenge for researchers to optimize the assembly of GO nanosheets.
Featured by great fracture toughness, natural abalones have the following properties: (1) grow at room temperature; (2) have a layered hierarchical nano-/microscale structure; (3) have abundant interface interactions. Inspired by the above features, the team of Prof. Cheng proposed to promote the interface interaction between adjacent graphene nanosheets through the design of different types of interface, so as to obtain graphene films with better physical and chemical performance. The large-area sp2 conjugated network provides abundant sites for dense π-π bonding, which contributes to a stronger π-π bonding. Besides, π-π bonding has the advantage of preserving the π-conjugated structure of graphene, which is beneficial to the charge transfer and tensile strength. However, due to the fact that the π-π bonding agents are small molecules, which greatly restrict the slippage of graphene nanosheets, the performance improvement is greatly moderated.
Herein, the research team of Prof. Cheng demonstrates the synthesis of ultrastrong, supertough, and highly conductive graphene films using a rationally designed long-chain π-π bonding agent to bridge rGO nanosheets derived from low-temperature oxidized GO precursors. The π-π bonding agent is a type of highly π-conjugated long-chain polymer composed of bis (1-pyrene methyl) docosa-10,12-diynedioate (BPDD, C16H9CH2OOC(CH2)8C≡C−C≡C(CH2)8COOCH2C16H9) monomers, which can not only bond neighboring graphene nanosheets by π-π interactions but also polymerize by 1,4-addition reaction of their diacetylene groups. The resulting π-bridged rGO (πBG) films exhibit an ultrahigh tensile strength of 1,054 MPa and a prominent toughness of 36 MJ/m3, which are higher than all the other records that have been published so far. Besides, they also have excellent electrical conductivity of 1,192 S/cm, which is comparable to the graphene films made in an high-temperature process.
With an enhanced alignment graphene nanosheet alignment owing to long-chain π-π bonding, the resulting π-bridged rGO (πBG) films have extraordinary electromagnetic interference shielding effectiveness (EMI SE). In addition, the πBG film also exhibits excellent fatigue resistance and shows remarkable retention of mechanical and electrical properties during cyclic film stretch and film folding. More importantly, Raman measurements and molecular dynamics (MD) simulations together demonstrate the highly efficient stress transfer and large slippage of graphene nanosheets that cause improvements in both tensile strength and toughness.
The fabrication process of πBG films is displayed in Figure 1A. First, GO nanosheets are assembled into a GO film by vacuum filtration. The GO film is then reduced to produce an rGO film using HI. Finally, the BPDD is infiltrated into the rGO film and polymerized using UV radiation, resulting in a πBG film. A resultant flexible silver-gray πBG film is shown in Figure 1B and an ordered layered structure can be observed from the scanning electron microscopy (SEM) images (Figures 1C and 1D). Furthermore, the degree of graphene nanosheet orientation is quantified by wide-angle X-ray scattering (WAXS) patterns (Figures 1E and 1F). The result proves that the platelet of πBG films is better aligned compared with that of the rGO films.
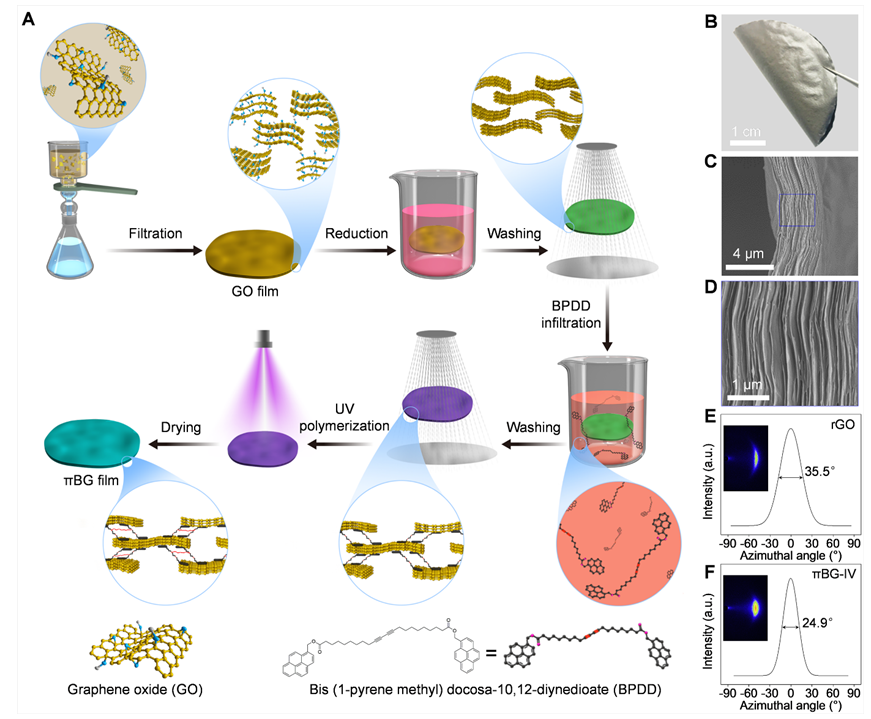
Figure 1. Fabrication and Structural Characterization of πBG Films
The typical tensile stress-strain curves of πBG films are shown in Figure 2A. The mechanical properties of πBG films can be optimized by adjusting polydiacetylene contents. According to Figure 2B, as a result of long-chain π-bridging, the tensile strength and toughness of πBG-IV film are substantially improved to 1,054.2 ± 56.5 MPa and 35.8 ± 1.9 MJ/m3, respectively, which are 2.9- and 4.6-fold higher than for rGO film. To our knowledge, the tensile strength, toughness, and electrical conductivity of πBG-IV film are the highest reported values for graphene films bridged through various bonding types under ambient environment (Figure 2C). Reflecting higher electrical conductivity and higher rGO nanosheet alignment, the πBG film (∼36.5 dB) has a much higher average EMI SE in the frequency range between 0.3 and 18 GHz than rGO film (∼24.9 dB) with similar thickness, as shown in Figure 2D. According to Figures 2E and 2F, absorption is the dominant shielding mechanism in πBG films. Moreover, compared with other solid shielding materials, πBG films have better overall shielding effectiveness, as proved in Figure 2G.
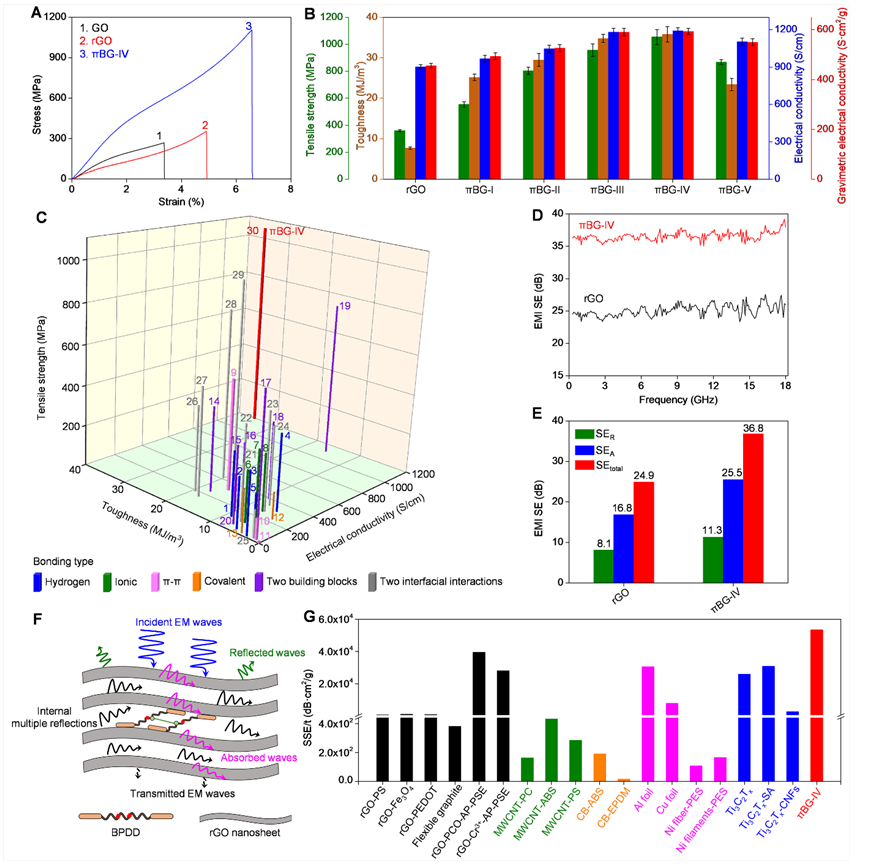
Figure 2. Properties of πBG Films
As displayed in Figure 3A, the πBG-IV film also exhibits better fatigue resistance than rGO film. More specifically, the rGO film can only be stretched for 41,683 cycles at a stress range between 190 and 260 MPa, while the πBG-IV film can be stretched for 264,811 cycles at a stress range between 780 and 860 MPa, as shown in Figure 3B. Due to the prominent fatigue crack suppression, the πBG-IV film has higher stability in mechanical and electrical properties, and EMI SE during repetitive tensile stretch, than rGO film. As shown in Figure 3C, the property retention percentages for tensile strength, electrical conductivity, and EMI SE of the πBG-IV film are 93.4%, 85.3%, and 89.3% after 100,000 stretch cycles between 160 and 240 MPa, respectively. More specifically, the tensile strength, electrical conductivity, and EMI SE of πBG-IV film repeatedly folded for 1,000 cycles retain 81.2%, 78.4%, and 84.1% of their original values, respectively.
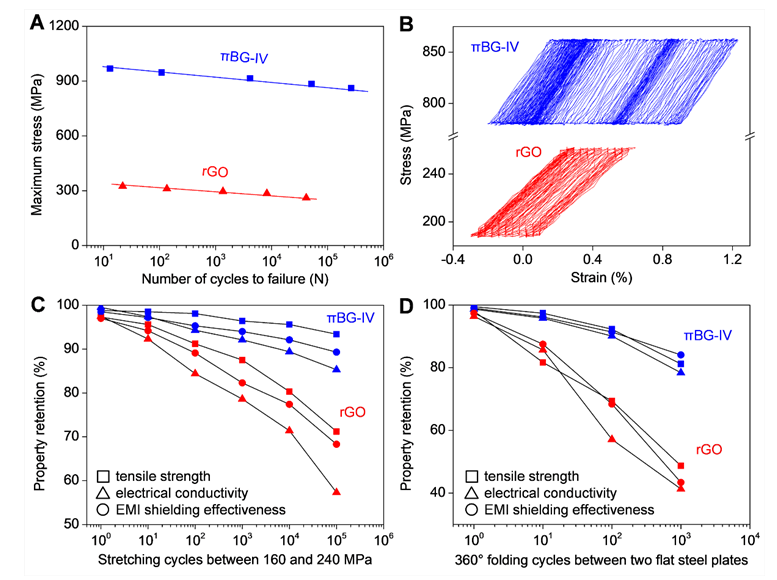
Figure 3. Property Stability of rGO and πBG-IV Films during Repetitive Tensile Stretching and Folding
The strain dependence of the graphene G-band frequency during in situ Raman testing visually demonstrates the upper strain limit of the plateaus for πBG-IV (Figure 4B) is larger than that of rGO (Figure 4A). These results indicate that the long-chain polydiacetylene molecules can provide much more movement space for rGO nanosheets, therefore causing the increase in the strain to failure. Interestingly, the simulative tensile stress-strain curves for both rGO and πBG-IV films are similar to those measured in our experiments, as shown in Figure 4C. The model snapshots for πBG-IV film during simulative tensile stretching are shown in Figure 4D. Upon initial stretching, the crumpled rGO nanosheets are first aligned and straightened. The coiled polydiacetylene (represented by dimeric BPDD) molecular chains are then gradually stretched with continuous loading to provide enough movement space for rGO nanosheets. When the loading is further increased, the stretched polydiacetylene molecules are pulled out and separated from the surface of the rGO nanosheets. A corresponding cartoon of the fracture process of πBG-IV film is shown in Figure 4E, which matches well with the strain dependence of the Raman frequency shift. In addition, the SEM images (Figures 4F and 4G) of fracture surfaces show curling of platelet edges for the πBG films, but not for the rGO film, which further demonstrates the strong interplatelet π-π bridging.
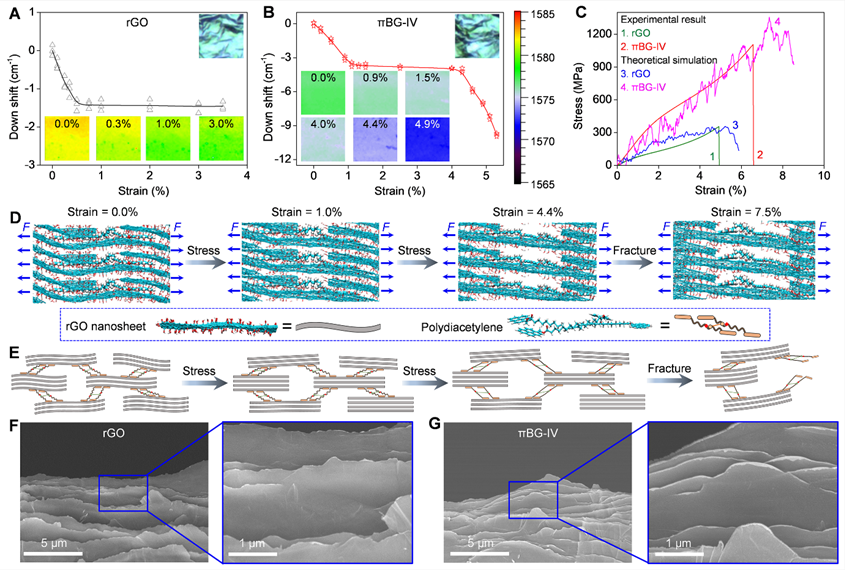
Figure 4. Fracture Mechanism of πBG Films
The graphene film bridged by long-chain π-π bonding agent proposed by the team of Prof. Cheng has promising applications in creating materials for use in the fields of aerospace and flexible electronics, which offers implication for the fabrication of graphene nanocomposites with high properties.
The research is supported by Outstanding Youth Science Foundation, the National Natural Science Foundation of China, Program of Introducing Talents of Discipline to Universities, Beihang University and so on. Other scholars who contribute to the research article include Chen Ying, a PhD student from Peking University Hospital of Stomatology, Dr. Wang Yanlei from Institute of Process Engineering, Chinese Academy of Science, Prof. Jiang Lei, Prof. Liu Yuzhou and Li Guangwen from the School of Chemistry, Beihang University, Prof. Xu Zhiping from Tsinghua University, Zhang Jianqi, Liu Luqi and Dr. Wang Guorui from National Center for Nanoscience and Technology as well as Prof. Antoni P. Tomsia, Chief Scientist of Advanced Innovation Center for Biomedical Engineering.
The article is available at :
https://www.cell.com/matter/fulltext/S2590-2385(19)30022-0.
More information on the website of the research team of Prof. Cheng Qunfeng:
http://chengresearch.net/zh/home-cn/.
Reported by Wang Jingjuan
Edited by Jia Aiping
Reviewed by Li Hongjie
Translated by Xiong Ting